Nobel Lecture: Jennifer Doudna, Nobel Prize in Chemistry 2020 | Summary and Q&A
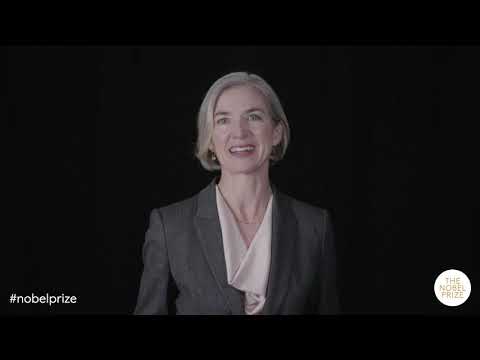
Summary
In this video, Jennifer Doudna discusses the science behind CRISPR-Cas9 as a genome editing technology. She explains the origin of the CRISPR system in bacteria and how it acts as an adaptive immune system. Doudna describes the three steps of CRISPR acquired immunity: adaptation, expression, and interference. She then delves into the discovery of CRISPR-Cas9's function as a dual RNA-guided protein and how it can be engineered to target specific DNA sequences. Doudna highlights the potential applications of CRISPR-Cas9 in correcting disease-causing mutations and the ethical considerations surrounding germline editing. She also discusses the diversity of CRISPR systems in nature and the future possibilities for CRISPR technology.
Questions & Answers
Q: What is the origin of the CRISPR system?
The CRISPR system originated in bacteria as an adaptive immune system to protect against viral infection. It allows bacteria cells to acquire small pieces of viral DNA into the CRISPR locus in their genome and use that information to detect and cleave viral DNA using CRISPR-associated proteins.
Q: How does CRISPR-Cas9 function as a genome editing technology?
CRISPR-Cas9 is a dual RNA-guided protein that can be programmed with single guide RNAs to target specific DNA sequences. When a guide RNA matches a sequence in the DNA, Cas9 cuts the DNA, triggering repair mechanisms in the cell. This repair process can introduce desired changes to the genome, allowing for precise genome editing.
Q: What are the three steps of CRISPR acquired immunity?
The three steps of CRISPR acquired immunity are adaptation, expression, and interference. Adaptation involves integrating small pieces of viral DNA into the CRISPR locus of the genome. Expression refers to the process of making RNA copies of the integrated viral sequences. Interference is the stage where RNA-guided detection of viral DNA occurs and Cas proteins cleave the viral DNA, providing protection to the cell.
Q: How did the collaboration with Emmanuel Charpentier and Christophe Chylinski contribute to the understanding of CRISPR-Cas9's function?
The collaboration with Emmanuel Charpentier and Christophe Chylinski helped answer the question of the function of the protein Crispr-Cas9. Their research showed that Crispr-Cas9 is a dual RNA-guided protein that uses a Crispr RNA molecule and a tracer RNA molecule to guide Cas9 to target DNA sequences. This finding allowed for the engineering of a simple two-component system for directing DNA double-stranded cutting.
Q: How was the agency to program Cas9 with single guide RNAs discovered?
Martin Yanick, working in collaboration with Jennifer Doudna and Christophe Chylinski, discovered that Cas9 can be programmed with single guide RNAs. By designing guide RNAs that recognize different target sequences in a plasmid DNA, they demonstrated that Cas9 could cleave the DNA at the desired sequences. This breakthrough enabled the programmability of Cas9 for precise genome editing.
Q: How are double-stranded breaks in DNA repaired in eukaryotic cells?
In eukaryotic cells, double-stranded breaks in DNA can be repaired through two main pathways. The non-homologous end-joining pathway can sometimes introduce small disruptions to the DNA sequence, while the homology-directed repair pathway can integrate DNA with homology to the flanking sequence of the break. Cas9-triggered breaks can stimulate these repair pathways, leading to changes in the genome.
Q: What challenges and opportunities are associated with genome editing in somatic and germ cells?
Genome editing in somatic cells, which are fully differentiated cells, can correct mutations but is not heritable. In contrast, genome editing in germ cells, such as sperm or egg cells, has the potential to introduce heritable changes. While somatic cell editing offers opportunities in disease correction, germ cell editing raises ethical and societal concerns, especially in the context of human germline editing. Responsible use and transparency are vital in these areas of research.
Q: How could CRISPR-Cas9 be applied in the correction of disease-causing mutations?
The precision of CRISPR-Cas9 makes it a promising tool for correcting disease-causing mutations. One example is the correction of sickle cell anemia-causing mutations. By using Cas9 to edit the specific mutation, researchers have shown that this technology can effectively and safely correct genetic diseases.
Q: What are some potential future applications of CRISPR technology?
The applications of CRISPR technology extend across various areas, including public health, agriculture, and biomedicine. In addition to disease correction, CRISPR technology can be used for detection and specificity in viral testing. The versatility and programmability of CRISPR systems open up countless possibilities for future innovations in fundamental research and practical solutions to real-world problems.
Q: What are the challenges and future prospects for CRISPR technology?
Challenges in the future of CRISPR technology include improving delivery methods for CRISPR-Cas9 and related proteins into different cell types, particularly for human patients. Additionally, ongoing fundamental research is crucial for expanding our understanding of the diversity and potential of CRISPR systems. Responsible use of CRISPR in human germline editing is essential, and international efforts are underway to establish guidelines and criteria for its use. Collaboration, transparency, and careful consideration of ethical implications will continue to shape the future of CRISPR technology.
Takeaways
CRISPR-Cas9 has revolutionized genome editing by offering a precise and programmable tool for manipulating DNA. It originated from the adaptive immune system of bacteria and has been harnessed as a technology for genome editing in various organisms. The ability to program Cas9 with single guide RNAs enables targeted cleavage of specific DNA sequences. CRISPR technology holds immense potential for correcting disease-causing mutations, but it also raises ethical considerations, particularly in human germline editing. Future advancements involve improving delivery methods, exploring the diversity of CRISPR systems, and applying CRISPR technology for detection, specificity, and real-world applications in human health and the environment. Responsible use, transparency, and collaboration are essential for the ethical and effective use of CRISPR technology.
Share This Summary 📚
Explore More Summaries from Nobel Prize 📚
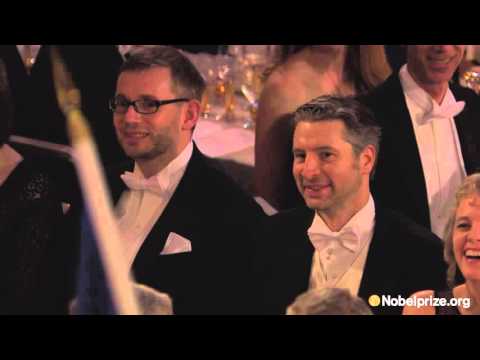
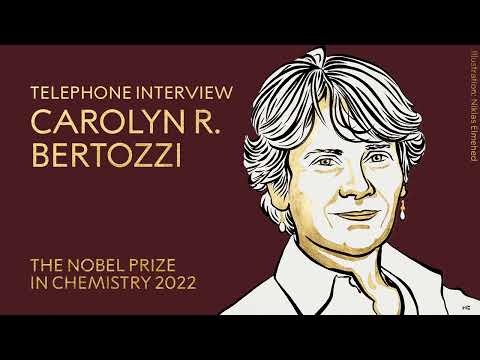
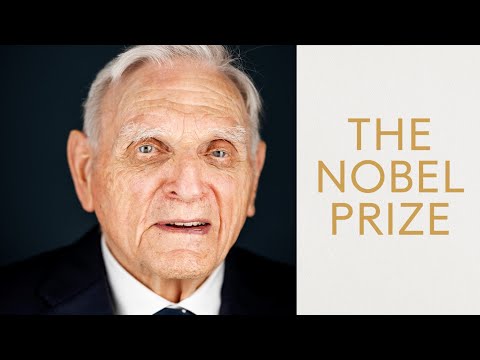
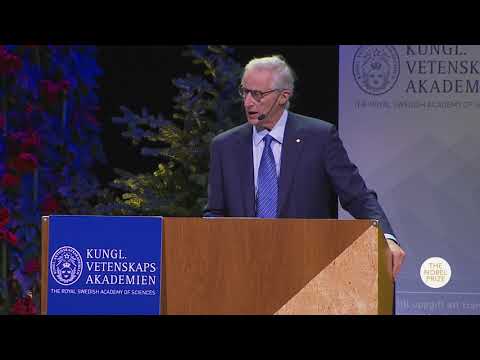

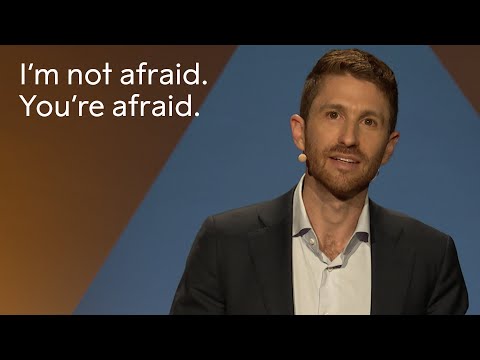